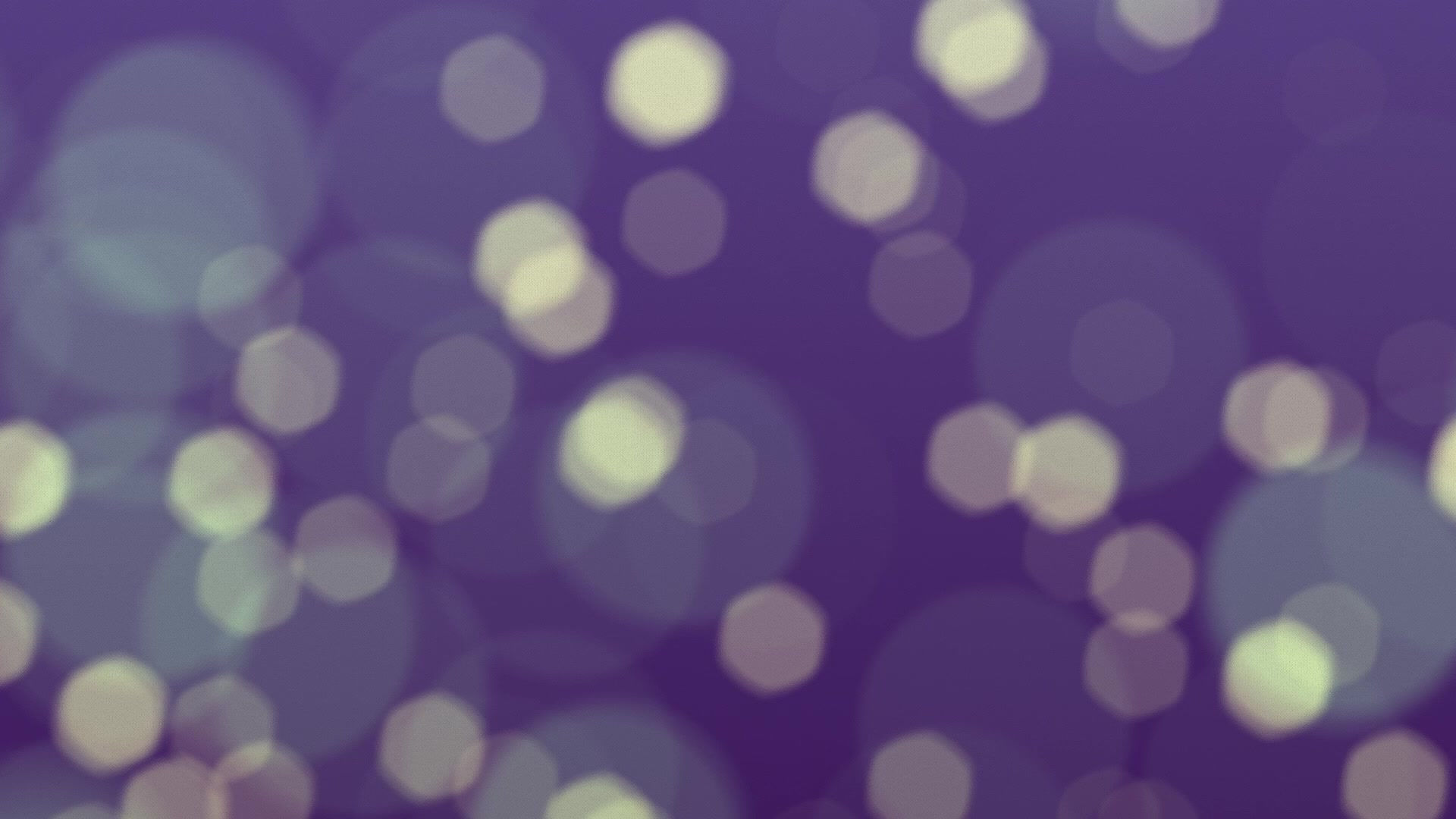
ANTIBIOTICS
Antibiotics, also called antibacterials, are a type of antimicrobial drug used in the treatment and prevention of bacterial infections. They may either kill or inhibit the growth of bacteria. A limited number of antibiotics also possess antiprotozoal activity. Antibiotics are not effective against viruses such as the common cold or influenza, and their inappropriate use allows the emergence of resistant organisms.
Antibiotics are a class of medication that combat infections and the diseases they cause (Campbell 2007). The term antibiotic is technically used for the natural products of the fermentation of special microorganisms. During fermentation the organisms produce the antibiotic material, which can then be isolated for use as a drug.
The term antimicrobials include not only antibiotics, but also synthetically formed compounds. In clinical practice, the terms antibiotic and antimicrobial are now used interchangeably (Scott 2009).
Antibiotics have revolutionised medical care and have had a significant role in reducing morbidity and mortality from diseases that were once widespread and untreatable. As a result of antibiotic development, surgery and other medical interventions once likely to result in significant sepsis are considerably safer
(Campbell 2007, Frost 2007). However, despite the significance of antibiotics, the development of resistance mechanisms that have spread in several clinically important bacterial species now limits their effectiveness, which is a serious public health concern (Campbell 2007). A working knowledge of antibiotics requires an insight into microbiology and general pharmacodynamic principles.
Antibiotics can be derived from either molds or from dyes.There are various antibiotics available and they come in various different brand names. Antibiotics are usually grouped together based on how they work. Each type of antibiotic only works against certain types of bacteria or parasites. This is why different antibiotics are used to treat different types of infection.
Basic Mechanisms of Antibiotics – Action and Resistance Inhibition of Cell
-
Wall Synthesis (most common mechanism)
-
Inhibition of Protein Synthesis (Translation) (second largest class)
-
Alteration of Cell Membranes
-
Inhibition of Nucleic Acid Synthesis
-
Antimetabolite Activity
Inhibition of bacterial cell wall synthesis
Unlike prokaryotic cells, the eukaryotic cells of humans do not possess peptidoglycan or contain a cell wall. This makes the wall of the bacterial cell an ideal target for antibiotic therapy because the therapy will not target the human
cell (Hills 2010). Penicillins and cephalosporins (beta-lactam antibiotics) are examples of antibiotics that interrupt peptidoglycan synthesis. These antibiotics enter the bacterial cell and bind to enzymes known as penicillin-binding proteins. This results in the formation of a weak or deformed cell wall, which swells and then bursts (Karch 2008). Drugs that destroy the cell in this way have a bactericidal effect. No other antibiotic has been more important than penicillin, which was discovered by Alexander Fleming in 1928. The original penicillins include benzylpenicillin (penicillin G) and phenoxymethylpenicillin (penicillin V). Over time the structure of penicillin has been re-engineered
to develop semi-synthetic penicillins such as amoxicillin and flucloxacillin. The cephalosporins such as cefalexin and cefradine are currently subdivided into four generations as a guide to their relative activity against different bacteria. Between them, the penicillins and cephalosporins are effective against a wide range of Gram-positive and Gram-negative bacteria, and some anaerobic bacteria (Palit 2009).
Inhibition of bacterial DNA synthesis
The substance inside the plasma membrane of the bacterial cell is called the cytoplasm and is about 80% water. The nucleoid is a major structure in the cytoplasm and usually contains a single loop of DNA known as the bacterial chromosome.
This is the cell’s genetic information, which carries all the intelligence required for cell structure and function. Bacteria may also have DNA in separate loops within the cytoplasm known as plasmids. These molecules are not connected to the main bacterial chromosome and replicate independently. Plasmids can carry genes for activities such as antibiotic resistance (Frost 2007, Tortora et al 2010).
DNA replication and cell division are fundamental to the production of new bacterial cells, and some antibiotics work by inhibiting the manufacture of DNA. These antibiotics tend to be bactericidal in action and include the quinolones (such as ciprofloxacin) as well as drugs such as metronidazole, nitrofurantoin and rifampicin. Human cells also need to synthesise DNA, and so these drugs have to be designed carefully to achieve selective toxicity (Hills 2010). The quinolones inhibit the action of two enzymes, DNA gyrase and topoisomerase IV, that are essential for DNA replication. Damage to the DNA means the cell cannot be maintained, resulting in cell death (Karch 2008).
Rifampicin is a rifamycin antibiotic (derived from a bacterium called Nocardia mediterranei) which is mainly used to treat tuberculosis, but which has other indications such as adjunctive treatment in anti-staphylococcal therapy. It works by interfering with the manufacture of proteins that are essential to bacterial cell structure and function. Rifampicin achieves this by inhibiting the enzyme required for the formation of messenger ribonucleic acid (mRNA) (copies of the genetic code required to make new proteins within the bacteria cell). Resistance to rifampicin develops quickly an the drug should not be given as monotherapy, unless clinically indicated, for example in the prevention of a secondary case of bacterial meningitis (Simonsen et al 2006, Hills 2010). The antibiotic metronidazole uses a chemical reaction to disrupt bacterial DNA. The reaction occurs in the absence of oxygen, which means the antibiotic is effective only against anaerobic bacteria. Metronidazole is effective where oxygen levels are low, for example in treating Bacteroides spp. in peritoneal infections (Frost 2007).
Inhibition of bacterial protein synthesis
Both human and bacterial cells contain structures known as ribosomes located in the cell cytoplasm. The ribosomes that are responsible for protein synthesis are smaller in bacteria than in humans (Frost 2007). Antibiotics that inhibit protein synthesis act selectively in that they have a greater affinity to bacterial ribosomes than to those of humans (Simonsen et al 2006). Antibiotics that interfere with protein synthesis include the aminoglycosides, tetracyclines and macrolides (Hills 2010). The aminoglycosides (such as gentamicin) are bactericidal and cause misreading of the code on mRNA. This results in the bacteria creating proteins that are dysfunctional. Tetracyclines (such as oxytetracycline, doxycycline, minocycline and tetracycline) inhibit protein synthesis by blocking a molecule known as transfer RNA. This is the molecule that transports the amino acids essential for the manufacture of proteins. The macrolides (such as erythromycin and clarithromycin) bind to one of the ribosomal subunits and inhibit the ribosomes from functioning.
Inhibition of folate synthesis
Folate is essential for the manufacture of DNA (Hills 2010). In contrast to mammals that obtain folate from external sources (food), bacteria manufacture their own. Important antibiotics that inhibit folate synthesis include trimethoprim and the sulphonamides such as sulfadiazine. The sulphonamides are now rarely used as monotherapy because of growing resistance (Frost 2007, Hills 2010). Acting separately, sulphonamides and trimethoprim are bacteriostatic. Combined as co-trimoxazole, they work synergistically and are usually bactericidal. The sulphonamides have a poor side-effect profile including serious blood dyscrasias, notably bone marrow depression and agranulocytosis especially in older patients, and frequent rashes (British National Formulary (BNF) 2011). Therefore co-trimoxazole is usually restricted to infections where other options are not suitable (Hills 2010). However, it is still used as prophylaxis or treatment for Pneumocystis jirovecii (previously P. carinii) pneumonia seen in immunocompromised patients.
Mechanisms of antibiotic resistance Major mechanisms by which bacteria can develop resistance include:
-
The antibiotic can be destroyed or inactivated by the production of an enzyme. This mechanism of resistance mainly affects the penicillins and cephalosporins (Tortora et al 2010).
-
The antibiotic’s target molecule can adapt so the antibiotic no longer recognises and binds to the molecule. For example, changes in or absence of the penicillin binding proteins that are the receptor sites for beta-lactam antibiotics can result in drug resistance (Frost 2007, Finch 2009).
-
An antibiotic can also be inhibited from reaching its target site because the bacterial cell wall is modified, preventing the action of the drug. For example, some enterococci (Steptococcus spp. in the intestinal tract)have developed resistance to vancomycin in this way (Frost 2007).
-
Efflux resistance is a mechanism whereby proteins in the cell wall adjust to pump out the antibiotic so it cannot reach an adequate intracellular concentration. Resistance to the tetracyclines, macrolides and quinolones may be linked to efflux mechanisms (Frost 2007).
What is antibiotic resistance?
When bacteria develop the ability to defend themselves against the effect of an antibiotic, then they are said to have acquired antibiotic resistance. Over the years, pathogenic bacteria - the bacteria that cause disease - have grown resistant to many conventional antibiotics because of overuse or inappropriate use.
The emergence of resistant bacteria is associated with the widespread use of antibiotics. This is a threat to public health and especially to older patients and those who are debilitated or immunocompromised.
When To Use Antibiotics?
Antibiotics are specific for the type of bacteria being treated and, in general, cannot be interchanged from one infection to another. When antibiotics are used correctly, they are usually safe with few side effects.
However, as with most drugs, antibiotics can lead to side effects that may range from being a nuisance to serious or life-threatening. In infants and the elderly, in patients with kidney or liver disease, in pregnant or breastfeeding women, and in many other patient groups antibiotic doses may need to be adjusted based upon the specific characteristics of the patient, like kidney or liver function, weight, or age. Drug interactions can also be common with antibiotics. Health care providers are able to assess each patient individually to determine the correct antibiotic and dose.
When NOT To Use Antibiotics?
Antibiotics are not the correct choice for all infections. For example, most sore throats, cough and colds, flu or acute sinusitis are viral in origin (not bacterial) and do not need an antibiotic. These viral infections are “self-limiting”, meaning that your own immune system will usually kick in and fight the virus off. In fact, using antibiotics for viral infections can increase the risk for antibiotic resistance, lower the options for future treatments if an antibiotic is needed, and put a patient at risk for side effects and extra cost due to unnecessary drug treatment.
Antibiotic resistant bacteria cannot be fully inhibited or killed by an antibiotic, even though the antibiotic may have worked effectively before the resistance occurred. Don't share your antibiotic or take medicine that was prescribed for someone else, and don't save an antibiotic to use the next time you get sick.
Types of Antibiotics:
1)Penicillins - for example, phenoxymethylpenicillin, flucloxacillin and amoxicillin.
2)Tetracyclines - for example, tetracycline, doxycycline and lymecycline.
3)Cephalosporins - for example, cefaclor, cefadroxil and cefalexin.
4)Quinolones - for example, ciprofloxacin, levofloxacin and norfloxacin.
5)Lincomycins
6)Macrolides - for example, erythromycin, azithromycin and clarithromycin.
7)Sulfonamides and trimethoprim - for example, co-trimoxazole.
8)Glycopeptides
9)Aminoglycosides - for example, gentamicin and tobramycin.
10)Carbapenems
11)Chloramphenicol
Reference:
Roach - Introductory Clinical Pharmacology 7e (Lippincott, 2003)
Antibiotics: mode of action
and mechanisms of resistance
Kaufman G (2011) Antibiotics: mode of action and mechanisms of resistance. Nursing Standard.(25, 42, 49-55. Date of acceptance: February 10 2011.)
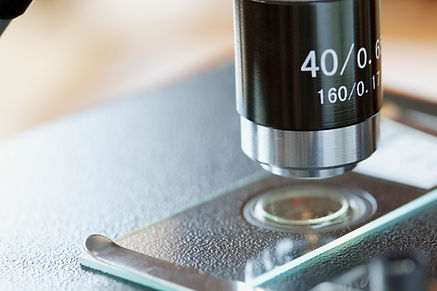
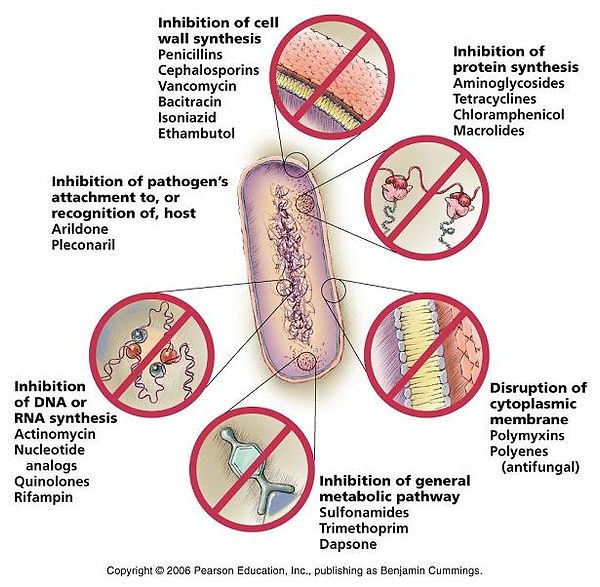